Next-Generation Antimicrobial Additives for Reactive Surface Coatings


There are more than 5,000 antimicrobial products, based on approximately 275 different active ingredients, currently registered and available in the U.S. marketplace. These antimicrobial pesticides are almost always organic chemicals and act by killing (biocidal) or inactivating microbes (biostatic). Antimicrobial pesticides have a wide array of applications in both public health-related products, for the control of those organisms infectious to humans, and products other than those related to public health, such as preserving agents in coatings, metal working fluids and wood supports.
Overlapping with the antimicrobial category are those materials that are considered biopesticides and are natural materials such as plant products (e.g., canola oil), bacteria (e.g., Pseudomonas fluorescens A506) and certain minerals/metals (e.g., sulfur or silver). By the end of 2005, there were approximately 232 active ingredients and 1057 products registered as biopesticides by the EPA. Generally considered safer to humans and the environment than conventional pesticides, the perceived advantages associated with biopesticides typically include a narrow target range, limited environmental persistence and a specific mode of action. While these characteristics may limit the risk of unintended consequences to either humans or the environment, they may also require either multiple applications, higher initial concentrations/doses or the use of multiple pesticides to achieve the desired degree of protection. The economic impact of efforts to control microbial pests is approximately one billion dollars each year1 (as measured in dollars spent on antimicrobial pesticides).

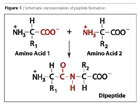
The Biological "Green" Approach: Antimicrobial Peptides
Reactive Surfaces, Ltd. faced a challenge in bringing biologically derived antimicrobial peptides into surface coatings - in particular, how best to preserve the antimicrobial nature of these biomolecules in the mileau of the coating. In another of its bio-engineered additive platforms, the company developed enzymatic activities for such coatings5-7, so the knowledge was there to make the attempt. The task was to identify antimicrobial peptides that would retain their biocidal activity in coatings; to identify peptides that could be relatively economically manufactured; to modify them as necessary to address the realities of the coating environment; to develop techniques that would allow rapid-throughput testing; and to test the additives in a variety of coatings and against a variety of target microorganisms in the development of the ProteCoat solution.In this two-part series, we describe the conceptual goals, experimental approach and initial proof-of-concept experiments demonstrating the feasibility of using ProteCoat as a biocidal additive in coatings. The second part of this series (October 2006) will describe results in a variety of coatings, using ProteCoat alone and in combination with existing biocides, and against a variety of target microorganisms. In addition, the engineering of coatings and peptides to maximize biocidal efficacy will be described.
The search for novel antimicrobials has been invigorated by the realization that humans, as well as most other multicellular organisms, live relatively amicably within a sea of microbes. To put this into perspective, consider that in the human body there are about 1013 cells - and 1014 bacteria. While most of these microorganisms live in the large intestine, there are approximately 103-104 microbes per cm2 on the skin, and a wide variety that live in the nose, mouth, stomach and small intestine. Molecular biology research from the past two decades suggests there may be as many as 4,000 - 13,000 different types of microbes in a teaspoon of soil, and 5 to 50 million in a teaspoon of ocean water. To maintain the advantage, the human body and its commensal population of microorganisms produce an arsenal of compounds including bacteriocidins, defensins and other cationic peptides, all of which work to keep invading microbes at bay. Antimicrobial peptides (AMPs) are present on mucosal surfaces (nasopharyngeal, respiratory, etc.), on the body surface, and within the granules of phagocytes. They have been identified and characterized from, among other sources, silk moth, frog, human, horseshoe crab, scorpion, pig, plant, monkey, radish, Drosophilia, and honeybee, and they provide the template for the next generation of "green" biocides.
Although a rigorous classification of these AMPs is difficult due to their wide diversity and generic functions, they are often characterized by their physical properties. In general, peptides are a family of molecules formed in nature by polymerization of biologically synthesized compounds known as amino acids. Amino acids are one of the basic structural building units of life and, as such, are either synthesized or scavenged by all known life forms to produce the amino acid polymers we know as proteins and peptides (Figure 1). Peptides are usually distinguished from proteins on the basis of length, and they perform a wide array of functions in living systems. For example, vasopressin is a signaling peptide composed of nine amino acids and it is found in humans and other organisms. It is released in response to signals that the body is low on water, and acts to conserve water by causing the kidneys to reduce urine volume. A more familiar peptide is the hormone insulin, which is composed of two peptide chains of 21 and 30 amino acids and is responsible for the maintenance of blood glucose levels.

In general, these cationic peptides have an enormous variety of sequences and structures. There are, however, certain features that are common, each of which was critical to designing active ingredient peptides for the ProteCoat additives. The natural cationic peptides are generally 12-50 amino acids in length, have a net positive charge, and contain around 50% hydrophobic amino acids. They assume a three-dimensional structure, or fold, due either to the presence of internal interactions between individual amino acids or because of contact with membranes. Nevertheless, both the folding (secondary structure) of the cationic peptides, and their amino acid sequences (primary structure), even within a given class of secondary structures, are quite heterogeneous. The four structural classes include b-sheet molecules with two or more intermolecular interactions (disulfide bonds between cysteine residues), linear molecules that fold upon insertion into the membrane into amphipathic a-helices, extended molecules, and loop structures held by one disulfide. The b-sheet and a-helical molecules are by far the most common in nature (Figure 2).

Commercial Approach and Feasibility
As the number of naturally occurring AMPs continues to expand, the detailed examination of their modes of action and potential as therapeutic agents has been made possible by the use of synthetic peptides. For example, synthetic peptide chemistry was used to establish the primary sequences of the cecropins, originally isolated from the giant silk moth, and to develop synthetic analogues that are more active, stable and that have a broader spectrum of activity.8 An outgrowth of the synthetic chemistry used to generate peptides, combinatorial chemistry was born in the early 1980s when Mario Geysen9 created the first combinatorial library by the simultaneous synthesis of diversified peptides. The use of combinatorial synthetic methods allows a very large number of molecules to be synthesized much more rapidly and at lower cost than traditional synthetic chemistry. The use of combinatorial libraries also offers an efficient methodology to identify novel function.Families of peptides are available (permission to use those in Reactive Surfaces' proof-of-concept studies was kindly granted by NCE Pharmaceuticals, Inc., San Diego CA), which have been developed from synthetic peptide combinatorial libraries (SPCL). Using a systematic evaluation of SPCLs, bioactive peptides have been identified that have been shown to be active against filamentous fungi. The NCE Pharmaceuticals peptides are novel (patented in the U.S. and abroad), biodegradable, have high efficacy against sporulating microorganisms, and are active against fungal and microbial pathogens without being toxic to humans, animals or the environment.10,11 Since toxicity to humans, as evaluated by lysis of red blood cells, appears to be influenced by both length of peptide and the strength of hydrophobic interactions12, the design template of the target peptides was only six residues. This adds further economic advantage, as the process of combinatorial synthesis followed by screening and selection could be limited to five iterations.11 This iterative process resulted in a 20-100x enhancement of antimicrobial activity, depending on the target organism (Table 2). As expected, these peptides exhibit no lysis of red blood cells, nor are they mutagenic.10

The integrity of the cell membrane can be evaluated using fluorescent stains, such as SYTOX Green. SYTOX Green stain is a high-affinity nucleic acid stain that does not cross the membranes of live cells and yet easily penetrates cells with compromised membranes. As can be seen by the localized appearance of SYTOX Green, the ethanol-treated spores (Figure 3A, microconidia) and hyphae (Figure 3C) have a compromised cell membrane, yet their DNA remains sequestered in the nucleus. For those conidia (Figure 3B) and hyphae (Figure 3D) that were visualized with SYTOX Green following treatment with the hexapeptide, the dye is diffuse throughout the cell, indicating that the cellular and the nuclear membranes have been compromised. Our observed experience is that all AMPs used in these studies are able to compromise exposed cellular membranes within 5 min, to cause rapid kill of spores and vegetative cells used to challenge the coated surfaces.
In the past, the only ways to obtain these small cationic peptides were to isolate them from the host organism, which required large amounts of material and yielded only very small amounts of peptide, or to synthesize them by chemical methods. One alternative available now is to use biological production processes to generate the peptides. Fermentation methods (for peptide production, successfully demonstrated over ten years ago13) will certainly be most economical as demonstrated with others of Reactive Surfaces, Ltd.'s bio-engineered additives (OPDtox™, see PCI, February 2005, pp. 26-33). AMPs have been successfully expressed using several genetic/biological approaches, including production as fusion proteins, inclusion bodies, repeats/multimers, or cell-surface expression. The advantages of producing these peptides in a bioexpression system include: (1) the relative ease with which the system can be scaled up: (2) cost effectiveness, and (3) the ability to make variants and utilize optimized isolation procedures. By developing and producing active peptides in the form found in nature, the peptides can be manufactured using existing fermentation technology, reducing production costs to acceptable commercial levels.
Having said that, the market for large-scale synthetic peptide production is also growing rapidly, fueled by industrial needs for various anti-microbial/anti-fungal peptides, pharmaceutical needs for peptides for human and animal therapeutics, as well as their use in cosmetics and as nutritional supplements. This large-scale chemical production of peptides can be achieved in a number of ways: solid-phase synthesis or solution-phase synthesis. Where manufacturing of research (gram) quantities of an antimicrobial peptide would be cost prohibitive, commercially feasible manufacturing of kilogram quantities of antimicrobial peptides is imminently achievable. While the actual sequence, length and any modifications present in the peptide will have dramatic effects on production costs, optimized synthetic processes will further dramatically reduce costs for large-scale peptide synthesis (personal communication, 21st Century Biochemicals, J. Fishman, Ph.D.).

ProteCoat™: Bio-Engineered Peptide Additives That Work in Coatings
In order to evaluate the concept of antimicrobial peptides as a biocidal coating component, the hexapeptide described above from the preliminary studies underwent further design considerations, resulting in a heptapeptide, AMP-7, of similar composition and activity (NCE Pharmaceuticals, Inc.). To evaluate the efficacy of ProteCoat additives when added to coatings, a dilution series of ProteCoat was added to UCAR 451 (Dow Chemical), a clear, styrene-acrylic latex. ProteCoat (Ver. NCEP-AMP-7) was dissolved in UCAR 451 and the ProteCoat-UCAR coating was applied to the bottom of 96-well clear polystyrene plates, and then challenged with an inoculum of 104 spores/ml of Fusarium oxysporum f. sp. lycopersici. The spores were subsequently transferred to a growth media, and allowed to grow for 48 hrs at 25 ºC. As expected, the MIC of the admixed ProteCoat was greater than that of the peptide ingredient in broth, with the pattern of growth inhibition in the coating determined the MIC to be 1.17 mg/ml. There was clear retardation of growth even at the lowest peptide concentration tested, which was 0.78 mg/ml, at the 24 hr time point (although in the presence of this high of a spore challenge, by 48 hrs this ProteCoat concentration was overwhelmed and the inhibition was ultimately less than 10%).As previously discussed, studies using the membrane-impermeable fluorescent stain SYTOX Green demonstrated that the parent AMP (FRLKFH) compromises the integrity of the cell membrane as well as the internal nuclear membrane surrounding the DNA.10 This is apparent in Figure 3 where the fluorescent reporter is concentrated in the nucleus (3A and 3C) of the chemically permeabilized spores and hyphae, and uniformly dispersed throughout the cell in the AMP compromised cells (3B and 3D). This technique has been adapted to provide a high-throughput screening for testing antimicrobial coatings (ProteCoat + UCAR 451), and suggest a similar mechanism. The ProteCoat-UCAR mix is painted onto the bottom of 96-well clear polystyrene plates as previously described, and then challenged with an inoculum of 107 spores/ml of Fusarium oxysporum f. sp. lycopersici). The addition of SYTOX Green allows the spores to be monitored for viability in real time. This assay reduces the time required to evaluate the antimicrobial efficacy of the coating to 3 hours. As the concentration of ProteCoat in the coating increases, the fluorescence increases as the cell becomes permeabilized and allows SYTOX Green to enter. At this stage, the SYTOX Green is localized in the nucleus. At higher peptide concentrations, the fluorescence decreases by approximately 25%. This occurs as the nuclear membrane is disrupted and the chromosomes become dispersed throughout the cell (Figure 4). It is of interest to note that the effect of the antimicrobial coating was relatively quick; there is no significant difference in the membrane permeability after 30 minutes than after 3 hrs (comparing the 30 min and 3 hr bar at each ProteCoat concentration). The MIC was determined to be between 1.6 mg/ml and 3.2 mg/ml, even though the coatings were challenged with 107 spores/ml, three logs more spores than in the growth studies. This level of spore challenge significantly exceeds the average ambient spore challenge (of 100 - 1,000 spores per cubic centimeter) by several orders of magnitude.
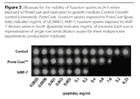

Why ProteCoat™ Biocidal Additives for Coatings?
ProteCoat additives offer substantial advantages over the traditional biocidal/biostatic chemicals presently used in coatings. They are biodegradable, work under mild conditions, provide safe alternatives to existing pesticides, and are not associated with the production or release of hazardous compounds to the environment. The antimicrobial ProteCoat additives described here bring a new technology to bear on the problems of certain existing biocides that are viewed as hazardous to the environment or to humans, offering an opportunity for protection without the adverse human-health and environmental impacts of the traditional approaches.Equally important is the adaptability of ProteCoat peptide-based additives. The diversity of antimicrobial peptides identified in nature provides a variety of design templates for tailoring a new generation of environmentally friendly antimicrobial additives for a wide variety of coatings. Combinatorial libraries of antimicrobial peptides provide a wealth of candidate biocides adaptable to specific coating requirements. With the rapidly increasing database of naturally occurring AMPs and the available recombinant DNA technologies, the tools exist today that will allow AMP additives to be developed for specific coating applications (e.g. architectural, technical paper, military, homeland security, in-can, in-process, on-surface, etc.).
By developing ProteCoat additives bioengineered to exhibit toxicity selective for microbes, and rapidly killing microorganisms over a broad antimicrobial spectrum, Reactive Surfaces is at the leading edge of this "green revolution" in biocide manufacturing. Since the ProteCoat additives are designed to work alone or synergistically with existing biocides, the use of the ProteCoat additives can be integrated with other biocidal products to enhance their activity. The large number of potential applications for these "green" biocides, including housing, surfaces in hospitals, schools, government facilities, industrial sites, and public transportation systems will expand the types of coatings into which the ProteCoat additives may be used. The development of cost-effective, antimicrobial surfaces will mitigate hazards for building occupants, decrease the time needed for reclamation of contaminated spaces and minimize environmental impacts commonly associated with traditional biocides.
For more information, contact Steve McDaniel at 512/472.8282, fax: 512/472. 8181, or e-mail smcdaniel@reactivesurfaces.com.
Looking for a reprint of this article?
From high-res PDFs to custom plaques, order your copy today!